Progress in particle physics relies on appropriate detection techniques. While over the last decades, a significant degree of standardisation has occurred for high-energy experiments, new detector approaches enabled by quantum technologies are increasingly opening up new parameter spaces in low-energy particle physics. At the same time, some of these technologies might also allow for higher sensitivity detectors at high energies.
Several attempts to explore the application of specific experimental techniques stemming from the fields of quantum optics, nanocrystals or 2-dimensional materials to experiments in high-energy physics have begun, while collaborations on topics relevant for low-energy particle physics approaches (with implications for BSM searches) in the areas of atomic interferometry, ultrasensitive cryogenic signal acquisition, and calorimetric detection of axions are being looked into.
Many developments stemming from advances in quantum technologies have opened up new parameter spaces for the search for BSM physics, with particular implications in searches for symmetry violations, searches for unknown interactions, searches for ultralight dark matter particles or fields, and precision determinations of masses or binding energies. In many cases, it is only the availability of a new technology that allows initially exploring and then subsequently systematically investigating these new parameter spaces, requiring the development of dedicated highly sensitive detection schemes. In many cases, technologies relevant to quantum computing or quantum communication form the basis of measurements sensitive to new fundamental physics or violations of symmetries. Reaching ultimate sensitivities often requires scaling such devices far beyond the initial proof-of-principle, an area in which expertise in designing, producing, and assembling large-scale devices — as is the case for high energy particle physics — may become crucial. At the same time, guidance from theory can help identify particularly interesting topics, can help avoid duplication, and indicate overlap with more sensitive searches that may have been carried out via other technologies.
Objectives
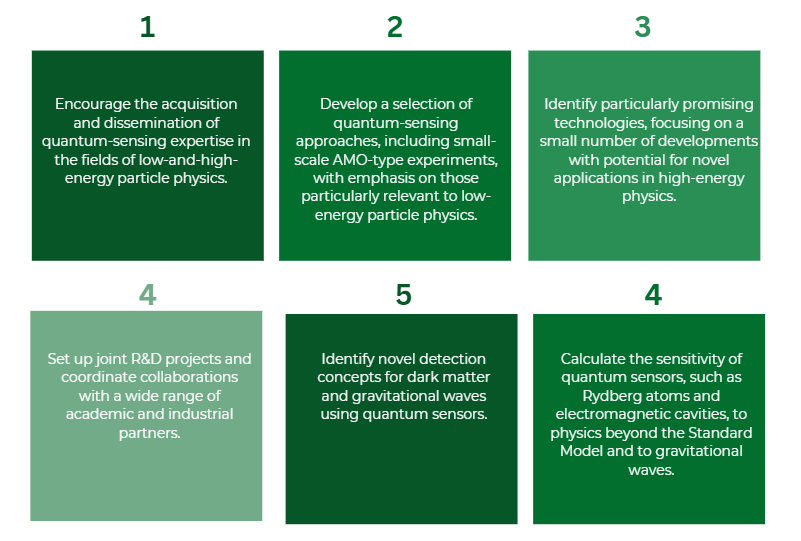
Current and future activities
The first project, which will shortly begin, investigates Graphene-based functional structures and nanostructures for novel gaseous detectors. The unique properties of two-dimensional materials such as graphene as well as carbon-based nanostructures including nanotubes, nanowires and quantum dots offer exciting possibilities for next-generation detector systems including wide-band photosensors. In the field of gaseous radiation detectors, these materials can be exploited to extend the sensitivity to wide-energy ranges of incident radiation, selectively influence charge production and transport processes and extend the longevity in challenging operational conditions. In the context of this doctoral student project in the CERN EP-DT Gas Detector Development team, we propose to pursue next-generation gaseous detector concepts taking advantage of novel materials and nanostructures. The research aims at exploiting graphene and nanomaterials for next-generation gaseous detectors including broadband gaseous photodetectors, robust precise timing detectors and graphene-enhanced ion-backflow suppression, which holds great potential for gaseous Time Projection Chambers (TPCs). In addition, potential synergies with other CERN groups working on graphene, Diamond-Like Carbon (DLC) and nanostructures will be explored.
The second project (which began at the end of 2020) is porting experiment control systems currently being developed in the field of quantum optics to experimental investigations of possible symmetry violations in neutral atomic systems containing antimatter: antihydrogen, antiprotonic atoms and positronium. The first two systems are only available at CERN's Antihydrogen Decelerator facility, whereas positronium can be obtained from radioactive sources even in small laboratories. For all three systems studies are ongoing or being prepared, benefiting from anti-hydrogen pulsed formation (2018) and long-lived positronium in Rydberg states (2015) and 23S metastable states (2019). Investigations with pulsed beams of positronium atoms of possible additional interactions, as well as possibly of 3-photon correlations in Ps decays, both using a novel approach (stemming from the field of atomic and molecular optics) for experiment control and data acquisition (Sinara), form the main thrust of the PhD project.
There is a large demand today for ultrafast timing detectors, and in particular for scintillator-based detectors. Particle physics experiments running at future accelerator facilities count on such fast-timing detectors to cope with the high event pileup due to the increase in luminosity and to enable particle identification capabilities. TOF techniques and event tagging with a minimum precision of 30ps can achieve pileup suppression by more than a factor of 10. The improvement of time resolution can be achieved with scintillators with a high photon density. Standard bulk scintillating materials are able to convert energy from high energetic impacts into optical photons at a rate of at most one photon per MeV per picosecond with approximately 50% efficiency which are nonetheless delayed by a risetime of the order of 100ps. This restricts the amount of information available within the first few picoseconds after the particle or gamma has interacted and is governed by the emission centre excited states decaying to their ground states with typical relaxation times of at least tens of ns. On the other hand, direct-band-gap-engineered semiconductor nanostructures show high potential for the emission of prompt photons due to quantum confinement. In particular, the potential of materials based on semiconductor quantum dots/quantum wells such as CdSe, InGaN/GaN or perovskite nanocrystals (eg. XPbBr3) as scintillator or charged particle tracking for HEP detectors will be explored.