Physics and high-energy physics are considered areas where quantum devices could make a difference in simulating complex dynamic interaction or multi-body systems.
Many classes of problems used in chemistry, condensed-matter physics or high-energy physics can be simulated through well-controlled quantum systems. In high-energy physics, quite a few possible applications have been investigated in recent years, especially in the context of gauge theories and their applications to dynamic problems (e.g. heavy-ion collisions), topological problems (e.g. CP violation), or high-baryon density configurations (e.g. modelling of neutron stars).
One possible approach is to design simulation strategies that apply different techniques, a mix of classic and quantum methods, to different parts of the problems and focus the attention on those areas that are computationally intractable using standard techniques. An example of this approach is the application of quantum circuits to describe the quantum properties of parton showers as a complementary technique to more classical Markov Chain Monte Carlo methods.
Many aspects of quantum devices can be understood rigorously using tools already well established in theoretical particle physics. By bringing together theoretical and experimental expertise, CERN can act as a catalyst for breakthroughs in quantum technologies and capitalise on expertise in the CERN Theory Department (CERN-TH).
Objectives
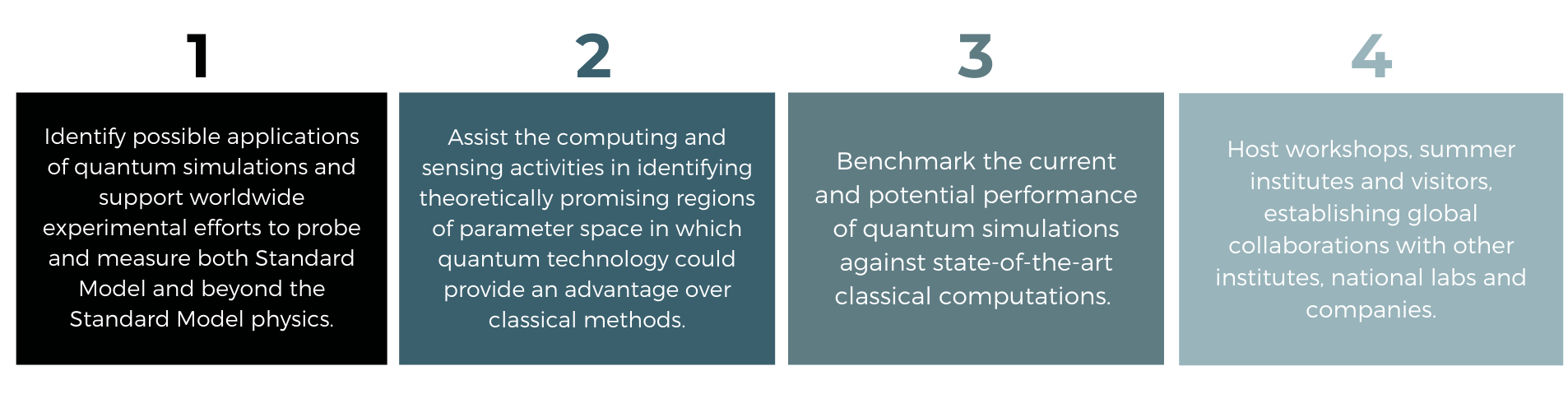
Current and future activities
Quantum field theories, by their nature, are rife with infinities. To connect theoretical predictions from QFT calculations to experimental results, these infinities must be dealt with via a process called regularization and renormalization. The only known generic method for doing so non-perturbatively is by implementing the field theory on a lattice, i.e. by discretizing space-time. An additional benefit of working on a lattice is that it allows one to simulate the theory on a computer, providing first-principle calculations of the properties of strongly coupled theories, including QCD.
This numerical simulation of Euclidean space-time correlation functions currently provides the only ab-initio method for extracting information about low-energy QCD/nuclear physics, with quantifiable errors. Current lattice QCD simulations allow for the measurement of light hadron masses, scattering parameters for certain few-body scattering events and the spectrum of several light hadrons. However, due to the limitations of Monte Carlo importance sampling, there are many questions that cannot be addressed with these tools, including the nature of the QCD phase diagram, particularly for large nuclear density, the real-time behaviour of quarks-gluon plasmas and even the masses of any but the lightest hadrons and nuclei.
The limitations of Monte Carlo also affect our ability to learn about the properties of high-energy QCD, including the structure of parton showers, as seen in LHC collisions. The Lattice Gauge Theory Simulation project will investigate if and how quantum devices and methods, rather than traditional Monte Carlo methods, may allow many of these questions to be explored. Another area taking centre-stage in physics research today is the physics of neutrino oscillation (periodic conversions of one neutrino flavour into another during propagation). The project Quantum Simulation of Collective Neutrino Oscillations will explore some of the least understood aspects of neutrino oscillations, as for example their dynamics in extreme environments such as supernova cores, where neutrino densities are so high that neutrinos influence each other so that the flavour evolution equations become highly non-linear. As this is intrinsically a quantum mechanical process, the goal of this project is to develop methods for simulating collective neutrino oscillations on a quantum computer.
Quantum machine learning (QML) algorithms are a promising approach to certain classes of applications in HEP phenomenology and theory, for example using variational quantum circuits and novel model architectures, which could benefit from the quantum hardware representation. The main motivation behind this planned activity is to identify the potential benefits of QML in HEP in terms of performance, precision, accuracy, and power consumption when compared to the current classical state of the art counterparts.
We will target applications on practical primitives for near-term quantum devices as well as advanced procedures for future, fully-fledged universal quantum computers. Research activities will include the development of hybrid classical-quantum models based on variational quantum circuit optimization and data re-uploading techniques, and the identification of new hardware designs which may accelerate QML performance. An important goal of this activity is the joint development of open-source libraries of quantum machine learning models designed for the hep-ph and hep-th applications based on open collaboration across the community. This activity is the natural bridge between the Theory and Simulation area and the Quantum Computing area of the CERN QTI.
Many developments stemming from advances in quantum technologies have opened up new parameter spaces for the search for BSM physics, with particular implications in searches for symmetry violations, searches for unknown interactions, searches for ultralight dark matter particles or fields, and precision determinations of masses or binding energies. In many cases, it is only the availability of a new technology that allows initially exploring and then subsequently systematically investigating these new parameter spaces, requiring the development of dedicated highly sensitive detection schemes. In many cases, technologies relevant to quantum computing or quantum communication form the basis of measurements sensitive to new fundamental physics or violations of symmetries. Reaching ultimate sensitivities often requires scaling such devices far beyond the initial proof-of-principle, an area in which expertise in designing, producing, and assembling large-scale devices — as is the case for high energy particle physics — may become crucial. At the same time, guidance from theory can help identify particularly interesting topics, can help avoid duplication, and indicate overlap with more sensitive searches that may have been carried out via other technologies.